Introduction to Phase-Matching
Phase-matching is a vital principle in nonlinear optics. It refers to a unique phenomenon where light waves of varying frequencies propagate harmoniously, maintaining a constant phase correlation to optimize energy exchange.
In the complex dance of light, phase-matching plays a lead role, choreographing a symphony of synchronization. It starts when an intense light wave passes through a nonlinear medium, initiating new waves at different frequencies. These waves must preserve phase coherence throughout their journey, ensuring they stay ‘in step’ to facilitate efficient energy interchange.
Achieving phase-matching involves offsetting natural dispersion in the nonlinear medium, where different frequencies tend to travel at distinct speeds. This process realigns the phase of these waves, optimizing energy transfer.
Phase-matching serves as the basis for several applications, including second harmonic generation and optical parametric processes. It ensures efficient interaction in nonlinear media, providing a solid foundation for applications in communications, laser technology, and cutting-edge research.
In the following sections, we’ll dive into the different types of phase-matching, its role in nonlinear crystals, and its myriad applications. Through this, we’ll appreciate how phase-matching drives innovation in modern optics.
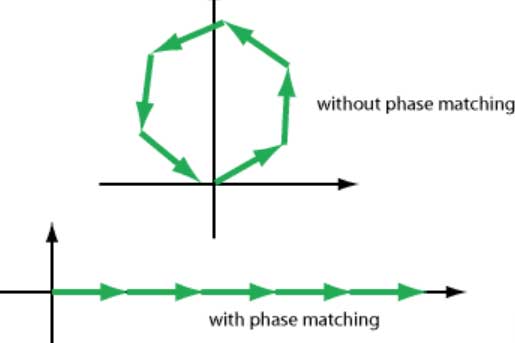
Understanding Nonlinear Crystals
In the realm of nonlinear optics, nonlinear crystals play a pivotal role. They are specialized materials that exhibit nonlinear optical properties, enabling light of one frequency to be converted into light of another frequency through a process known as frequency mixing.
Nonlinear crystals act as the primary medium for nonlinear optical processes. When an intense light beam passes through a nonlinear crystal, the interaction of the light with the crystal’s electrons leads to the generation of new light waves with different frequencies.
This frequency conversion process is based on wave mixing principles, where two or more waves combine to produce new waves. Phase-matching, which we discussed earlier, is a fundamental requirement for this process to occur effectively.
Not all materials can serve as nonlinear crystals. They must possess a high nonlinear coefficient, which measures how efficiently they can convert light from one frequency to another. Furthermore, they must also possess a large damage threshold to handle the high intensity of the light beams used in nonlinear optical processes.
Nonlinear crystals find application in various optical technologies. They’re used in frequency-doubling devices to generate green light from infrared lasers, in optical parametric oscillators for producing tunable light sources, and in quantum optics to generate entangled photon pairs.
In conclusion, nonlinear crystals are a cornerstone of nonlinear optics, providing the platform for many optical phenomena and applications. By understanding their properties and functions, we can better harness the power of nonlinear optics for technological advancement.
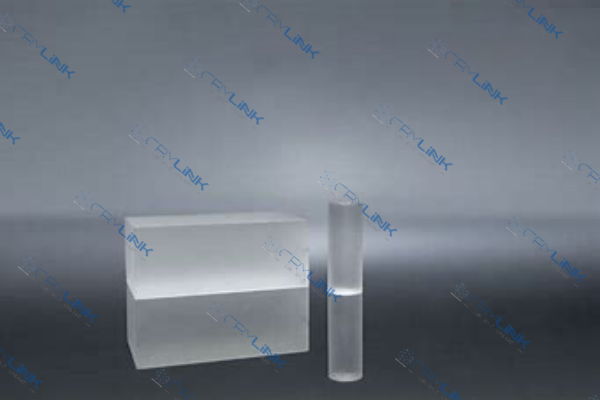
Types of Phase-Matching
Two fundamental categories of phase-matching are crucial in determining how effectively energy is exchanged in a nonlinear optical system: Type I and Type II phase-matching.
Type I Phase-Matching
Type I phase-matching, also referred to as ‘o-wave’ phase-matching, is a scenario in which all photons participating in the nonlinear optical process share the same polarization state. This type of phase-matching offers a potent advantage: it allows for an effective and efficient energy exchange due to the fact that the participating photons inherently share similar optical properties. This characteristic optimizes the phase-matching process, leading to a more efficient conversion of the optical frequencies.
Type I phase-matching is predominantly employed in nonlinear optical processes where maintaining the same polarization state for all the involved photons is imperative. These include but are not limited to processes such as second harmonic generation and optical parametric oscillation.
Type II Phase-Matching
Type II phase-matching is a contrasting scenario where the participating photons have orthogonal polarization states. Although it may appear that this would introduce challenges to the energy exchange process, Type II phase-matching offers a unique flexibility. It accommodates photons with different optical characteristics, thus widening the range of possible interactions and opening up opportunities for novel applications.
Type II phase-matching is often utilized in more complex nonlinear optical processes. For example, it finds use in parametric down-conversion, a quantum optical process where a photon decays into two photons with lower energies.
In conclusion, Type I and Type II phase-matching play significant roles in nonlinear optics. By understanding their distinctions and applications, we can harness their full potential, optimizing the energy exchange and broadening the application spectrum of nonlinear optical systems.
Quasi-Phase Matching
In the world of nonlinear optics, there are instances where perfect phase-matching may be challenging to achieve due to the intrinsic properties of the materials in use. This is where quasi-phase matching comes into play as an ingenious alternative.
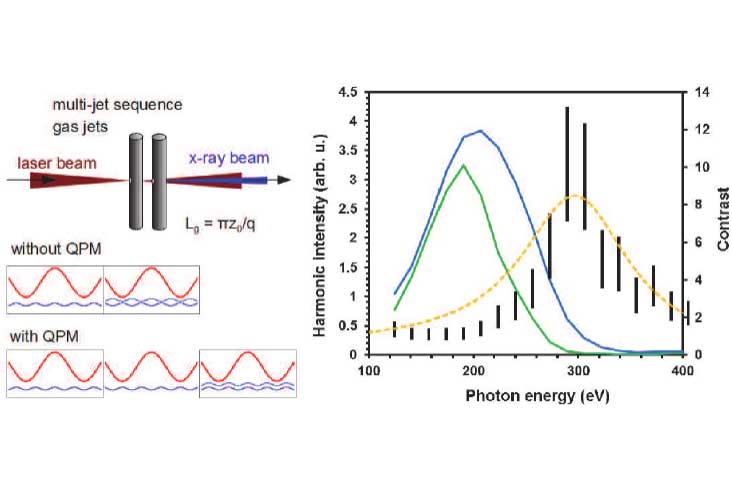
As the name suggests, Quasi-phase matching is a method to attain an “almost” phase-matched condition. It relies on a clever technique of manipulating the nonlinear coefficient of a medium in a periodic fashion. This process is commonly achieved by introducing a regular spatial modulation in the nonlinear optical susceptibility of the material. The purpose of this periodic modulation is to maintain phase coherence between the interacting waves, even in the absence of perfect phase-matching.
This innovative technique allows us to work around the limitations imposed by the natural dispersion characteristics of the medium. By periodically ‘resetting’ the phase relationship between the waves, it becomes possible to achieve efficient nonlinear interactions even in materials that would typically be deemed unsuitable for phase-matching.
Quasi-phase matching is particularly beneficial when high-frequency conversion efficiency is required in materials that exhibit strong dispersion. The utility of this technique spans across a multitude of applications, such as in the generation of high-power laser light or the production of entangled photon pairs in quantum optics.
Furthermore, quasi-phase matching allows for greater flexibility in the selection of the nonlinear medium. It opens the door to the use of materials that may offer other desirable properties, such as a higher nonlinear coefficient or superior thermal stability.
In conclusion, quasi-phase matching is an elegant solution extending nonlinear optics’ boundaries. It mitigates the challenges posed by natural dispersion and broadens the range of materials that can be effectively utilized, fostering further advancements in the field.
Critical Phase-Matching and Noncritical Phase-Matching
The pursuit of optimal phase-matching conditions leads us to the concepts of critical phase-matching and noncritical phase-matching. These terms describe the different strategies employed to achieve phase-matching, each with its unique set of benefits and challenges.
Critical Phase-Matching
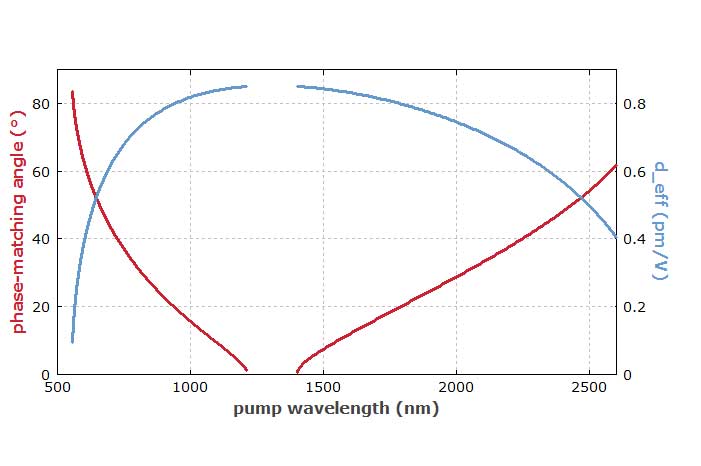
Critical phase-matching is a method that involves achieving phase-matching by altering the propagation direction of the light within the nonlinear crystal. This method is dubbed ‘critical’ due to its sensitivity to variations in the angle of light propagation. Even a slight change in this angle can disrupt the phase-matching condition, making it a delicate process to manage.
Despite its sensitivities, critical phase-matching offers the advantage of wide wavelength tunability. Simply adjusting the propagation angle makes it possible to achieve phase-matching for a broad range of input wavelengths. Critical phase-matching is particularly useful in applications such as tunable wavelength converters and optical parametric oscillators.
Noncritical Phase-Matching
Noncritical phase-matching, on the other hand, takes a different approach. It involves altering the temperature of the nonlinear crystal to achieve the desired phase-matching conditions. As the refractive index of the crystal changes with temperature, adjusting the crystal’s temperature allows for tuning of the phase-matching condition.
Noncritical phase-matching is less sensitive to angular deviations compared to critical phase-matching, making it a more robust option. This characteristic is advantageous in practical applications where maintaining a precise propagation angle may be challenging.
Both critical and noncritical phase-matching methods serve important roles in nonlinear optics. They provide unique pathways to achieve the desired phase-matching conditions and optimize the performance of nonlinear optical systems. By understanding the implications of each method, we can make informed decisions in designing and operating these systems for a wide range of applications.
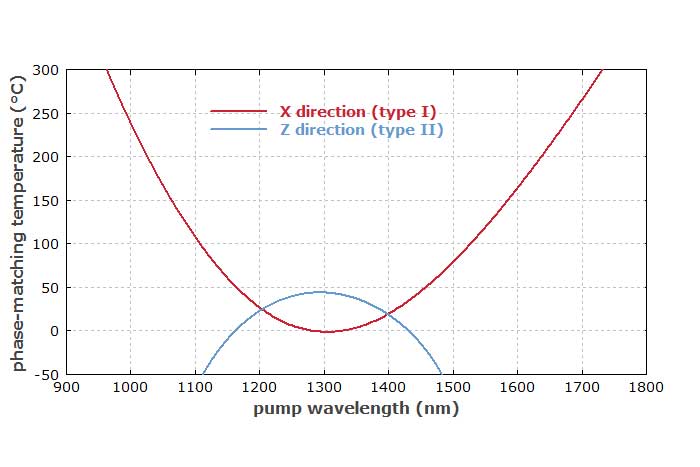
Angular and Temperature Phase-Matching
When we delve deeper into the mechanisms to achieve phase-matching, two techniques emerge as prominent strategies: Angular phase-matching and temperature phase-matching. These methods leverage the properties of the nonlinear crystal and the environment to create optimal conditions for phase-matching.
Angular Phase-Matching
Angular phase-matching refers to a method where the angle of incidence of light within the nonlinear crystal is adjusted to achieve phase-matching. As the refractive index in a crystal is dependent on the angle of light propagation, changing this angle can align the propagation constants of the interacting waves.
The crux of angular phase-matching lies in its precision. A slight variation in the angle can disrupt the phase-matching condition, making it a meticulous task to maintain. However, it offers a considerable advantage in terms of wavelength tunability. Adjusting the angle allows phase-matching across a broad spectrum of wavelengths, providing flexibility for various nonlinear optical processes.
Temperature Phase-Matching
On the other hand, temperature phase-matching takes advantage of the temperature dependence of the refractive index in certain crystals. By carefully controlling the temperature of the crystal, we can tune the refractive index to achieve the desired phase-matching condition.
Although it may seem more complicated than its angular counterpart, temperature phase-matching offers greater robustness. The phase-matching condition achieved through this method is less sensitive to minor variations, making it a more stable choice in practical settings where maintaining a precise angle or wavelength may be challenging.
In essence, both angular and temperature phase-matching play vital roles in nonlinear optics, providing unique pathways to achieve phase-matching and optimize the performance of nonlinear optical systems.
Applications of Phase-Matching
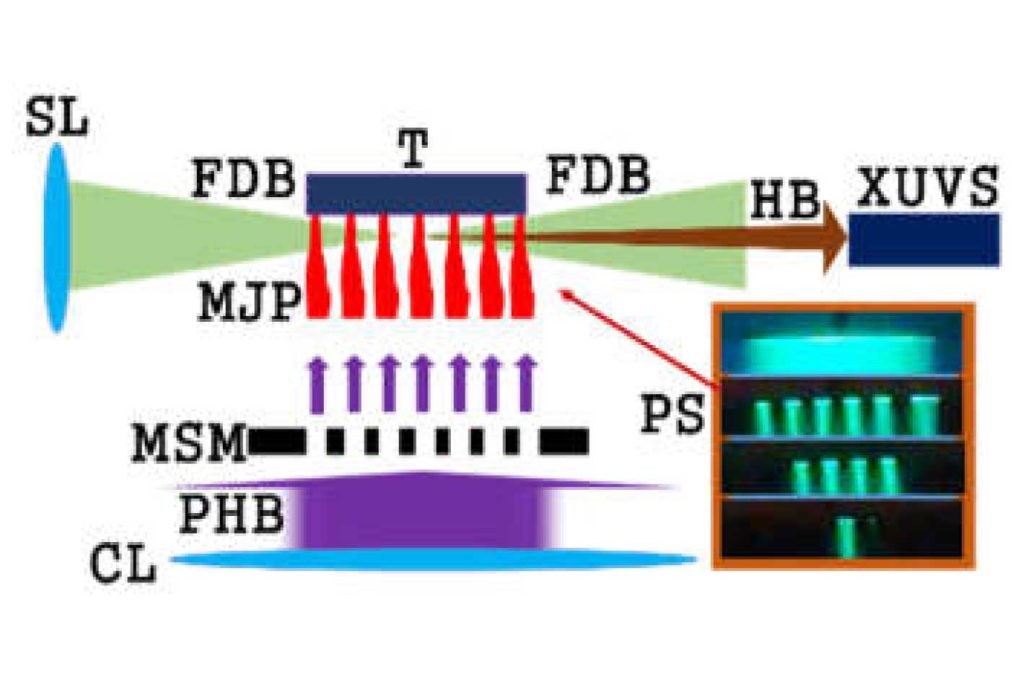
Phase-matching serves as the cornerstone of various applications in nonlinear optics, significantly contributing to advancements in communication, imaging, and scientific research.
One of the key applications of phase-matching is in the field of frequency conversion. Techniques such as second harmonic generation, difference frequency generation, and optical parametric oscillation all rely on phase-matching conditions for efficient conversion between frequencies.
Moreover, phase-matching plays an integral role in the development of tunable lasers. By leveraging the phase-matching techniques, lasers can generate light across a wide spectrum of wavelengths, serving critical roles in applications like medical imaging, spectroscopy, and even quantum communication.
Phase-matching is also fundamental to parametric down-conversion, a process vital for quantum optics and quantum information science. Ensuring the phase-matching condition makes it possible to generate entangled photon pairs, a resource central to quantum communication and computation.
Overall, phase-matching applications are vast and varied, demonstrating its crucial role in the evolution of optical technology and its potential to facilitate future innovation.
Conclusion
The different types of phase-matching are integral in exploiting the capabilities of nonlinear crystals. They facilitate energy exchange between light waves of varying frequencies, unlocking numerous applications in optics and beyond. Understanding these phase-matching types and knowing when to use them is the key to unlocking the full potential of nonlinear optical systems. The future of these systems, and indeed, nonlinear optics as a whole, hinges on continued research and innovation in phase-matching.