Introduction
High repetition rate solid-state lasers are at the forefront of laser technology, paving the way for a range of applications from materials processing to medical diagnostics. Central to their performance are the crystal materials used. In this article, we delve into the intricacies of diode crystals and ytterbium-doped crystals, analyzing their performance and implications in high repetition rate applications.
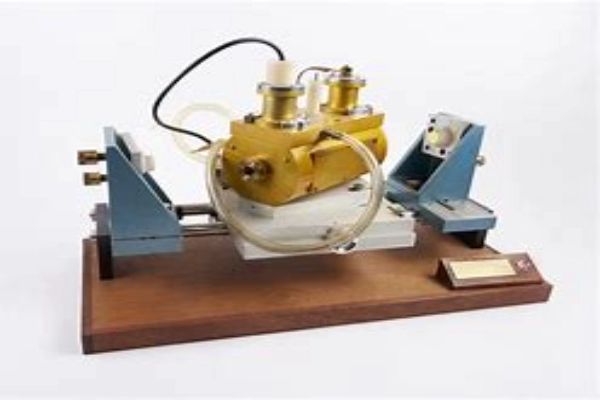
1. Basics of High Repetition Rate Solid State Lasers
Understanding the fundamental mechanics of high repetition rate solid-state lasers is essential for appreciating their capabilities and applications. At its core, the repetition rate of a laser is a pivotal parameter. It dictates the number of pulses the laser emits within a given timeframe, usually measured in seconds. So, when we discuss high repetition rate lasers, we’re referring to those laser systems that can achieve staggering rates, frequently exceeding a million pulses every single second.
This might prompt the question: Why is such a high repetition rate necessary? The answer lies in the vast spectrum of applications these lasers cater to. For instance, in precision machining or medical treatments, the ability to produce a large number of rapid pulses translates to unparalleled accuracy and efficiency. It means that these lasers can interact with materials or tissues with a precision that was once deemed impossible, making them invaluable tools in various scientific, industrial, and medical fields.
Central to the performance of these lasers is the crystal material employed in their design. Think of these crystal materials as the heart of the laser system – pivotal, indispensable, and responsible for the laser’s overall health. The intrinsic properties of these crystals, including their structural integrity, light amplification capabilities, and thermal conductivity, play a decisive role in determining the laser’s efficiency, power output, and operational lifespan.
Just like a human heart’s health can influence the overall wellbeing of a person, the quality of the crystal material can make or break a laser’s performance. Inferior or unsuitable crystal materials can lead to inefficiencies, reduced power, and even premature system failures. In contrast, top-grade crystals ensure that the laser operates at its peak, providing consistent and reliable performance even under the most demanding conditions. Given the pivotal role these materials play, ongoing research is continually striving to discover and optimize crystal materials to further enhance the capabilities of high repetition rate solid-state lasers.
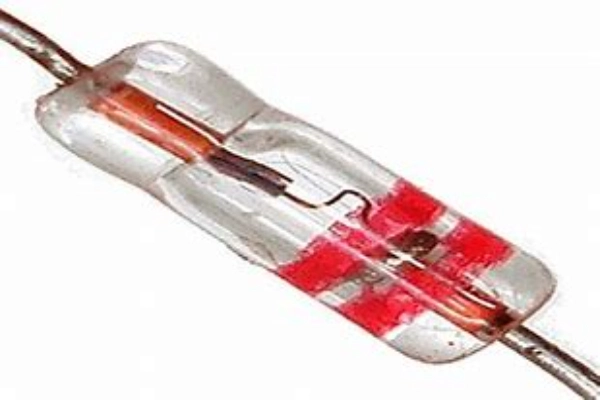
2. Dive into Diode Crystals
The world of laser technology is vast and varied, and at its forefront stand diode crystals, a class of materials that has significantly impacted the efficiency and performance of lasers. These are not your typical crystalline structures; they belong to the semiconductor family, which is known for its ability to conduct electricity under specific conditions. What sets diode crystals apart and makes them unique is their inherent ability to convert electrical energy directly into light, a feature pivotal for laser applications.
To understand the significance of this direct conversion, one must delve into the concept of a bandgap. Every semiconductor has what’s known as a bandgap – the energy difference between its conduction band and its valence band. Diode crystals possess a direct bandgap, which, in simple terms, means they have an aligned conduction and valence band. This alignment allows for the efficient emission of light without the need for excess energy or complex intermediary processes. When an electron transitions from the conduction band to the valence band in these crystals, it releases a photon, leading to light emission. This streamlined process is what makes diode crystals exceptionally suitable for laser applications, where efficient light generation is paramount.
However, the merits of diode crystals don’t stop at efficient light emission. One of the standout attributes of these materials, especially in the context of high repetition rate systems, is their efficiency. The physics and chemistry of diode crystals are such that they can maintain a high performance without compromising on their operational lifespan. Their inherent properties ensure that even when subjected to the demands of high repetition rate operations, they remain stable, reliable, and consistent.
Another critical factor that elevates diode crystals in the realm of high repetition rate laser systems is their thermal behavior. Heat generation can be a challenge in many laser systems, leading to inefficiencies and even potential damage. However, diode crystals are known for their low heat generation. This not only means less wear and tear but also reduces the need for complex cooling mechanisms, making the system more compact and cost-effective.
In the dynamic arena of laser technology, where performance and precision are non-negotiable, diode crystals have proven to be invaluable allies, pushing the boundaries of what’s achievable.
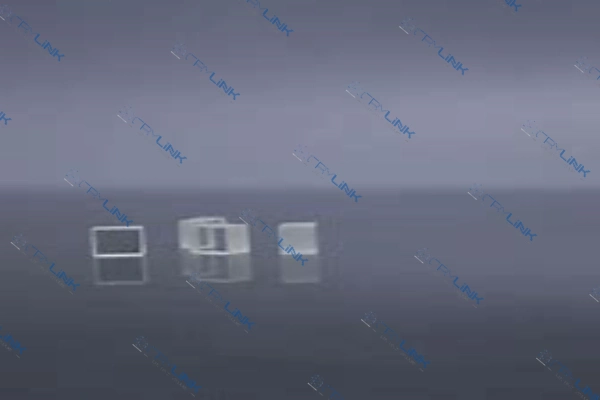
3. Understanding Ytterbium-Doped Crystals
In the intricate world of laser technology, the introduction of ytterbium-doped crystals has ushered in a new era of efficiency and performance. At its core, doping refers to the deliberate introduction of impurities into a material to enhance its properties. In the case of these crystals, ytterbium ions are introduced into the crystal lattice, which results in the amplification of light within the crystal.
Ytterbium-doped crystals have emerged as the materials of choice for many solid-state laser applications due to this unique capability. These crystals, when excited by a source, can amplify light remarkably, thanks to the ytterbium ions present within. These ions can absorb photons and, under the right conditions, release more photons than they absorb, leading to an amplified light beam.
When it comes to high repetition rate systems, these crystals exhibit several standout features that make them highly sought after. One of the most noteworthy is their broad gain bandwidth. In simpler terms, this means they can amplify a wide range of light frequencies. This broad bandwidth not only ensures versatility but also plays a pivotal role in maintaining the quality of the emitted beam.
Another attribute that adds to their appeal is their impressive thermal conductivity. As lasers function, heat generation is inevitable, and managing this heat is crucial for the longevity and efficiency of the system. Ytterbium-doped crystals, with their inherent high thermal conductivities, are adept at dissipating this heat, ensuring the system remains cool and efficient.
Furthermore, the structural uniqueness of these doped crystals ensures they can produce high power outputs. This power does not come at the cost of quality; even at elevated repetition rates, these crystals can maintain exceptional beam quality, making them invaluable in precision applications.
In conclusion, ytterbium-doped crystals, with their enhanced light amplification capabilities and other intrinsic properties, have set new benchmarks in the laser technology domain, paving the way for innovations and advancements.
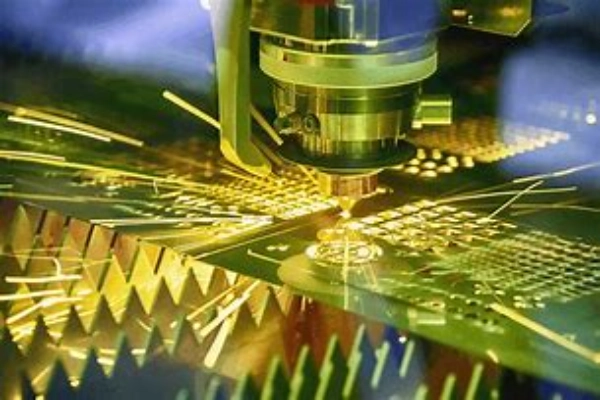
4. Comparative Analysis: Diode Crystals vs. Ytterbium-Doped Crystals
In the laser technology ecosystem, both diode crystals and ytterbium-doped crystals have established themselves as formidable players. Each comes with its own set of merits, making them ideal for specific applications. But how do they fare when pitted against each other?
When assessing efficiency and power output, a clear distinction arises. Diode crystals are renowned for their ability to efficiently convert electrical energy into light, making them stand out in applications where energy conservation is a priority. On the other hand, ytterbium-doped crystals take the lead in power output. Their inherent structure and the addition of ytterbium ions enable them to produce amplified light beams, making them particularly valuable in pulsed operations where high power is paramount.
Thermal management is yet another arena of competition between the two. Given their semiconductor nature, diode crystals naturally excel in dissipating heat, thereby reducing the chances of system overheating. This inherent ability translates to fewer cooling requirements and thus, a more streamlined system. Ytterbium-doped crystals, while inherently good conductors of heat, might require additional cooling mechanisms, especially in high-power applications. However, with the right infrastructure in place, they can match the performance of diode crystals.
Discussing longevity and durability, it’s essential to recognize that both materials are built for resilience. They have been used in various applications and have consistently proven their mettle in long-term operations. However, a blanket statement about their lifespan would be misleading. The durability of these materials can vary based on the specific laser application, operating conditions, and maintenance practices. In certain scenarios, diode crystals might outlast ytterbium-doped ones, while in others, the latter might have a more extended operational period.
In essence, the choice between diode crystals and ytterbium-doped crystals is less about superiority and more about suitability. Depending on the application and desired outcome, one might be favored over the other, underscoring the importance of understanding each material’s strengths and limitations.
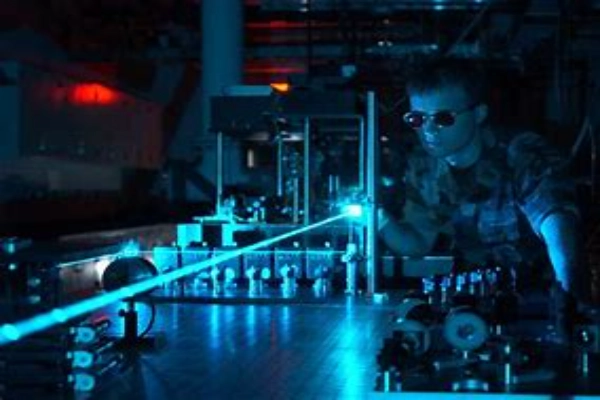
5. Application Insights
High repetition rate lasers, with their incredible speed and precision, have paved the way for groundbreaking applications across diverse sectors. Their capabilities have not only enhanced existing processes but also enabled novel approaches, ensuring better outcomes.
In the industrial sector, these lasers have revolutionized materials processing. Imagine the intricate designs on a piece of jewelry or the precision cuts on a metallic component; behind many of these processes is a high repetition rate laser. Their ability to produce rapid pulses ensures that materials like metals, ceramics, and even some polymers are processed with impeccable accuracy. This precision is particularly vital in industries like aerospace or electronics, where even the slightest error can have significant repercussions.
The medical field too has witnessed a paradigm shift with the introduction of these lasers. Procedures that once required extensive recovery times or resulted in significant scarring are now performed more cleanly and efficiently. Consider skin resurfacing treatments, where lasers help in removing damaged skin layers, stimulating collagen production, and leading to rejuvenated skin. Similarly, in surgical applications, these lasers can make fine incisions, minimizing tissue damage and promoting quicker healing for patients.
But the influence of high repetition rate lasers isn’t just confined to industries and hospitals. They have also made significant inroads into the world of research and development. Scientists, in their quest to understand the mysteries of the universe, often require tools that can operate at the limits of what’s technically possible. These lasers fit the bill perfectly. In fields like spectroscopy, they allow researchers to study the interactions between matter and radiated energy. Meanwhile, in microscopy, they enhance the resolution and clarity, enabling scientists to observe phenomena at incredibly minute scales.
Across these applications, one common theme emerges: the quest for betterment. Whether it’s creating a more resilient industrial component, ensuring a patient’s swift recovery, or unveiling the secrets of a novel material, high repetition rate lasers stand as testament to human ingenuity and the endless pursuit of progress.
Conclusion
Exploring the depths of diode crystals and ytterbium-doped crystals provides valuable insights into the world of high repetition rate solid-state lasers. Both materials have their merits, making them indispensable in the ever-evolving landscape of laser technology. As we continue to harness their potential, we are poised to unlock new applications and innovations in various domains.
FAQs
- What is the primary difference between diode crystals and ytterbium-doped crystals? Diode crystals are semiconductors, while ytterbium-doped crystals are solid-state laser materials enhanced with ytterbium ions.
- How does the repetition rate influence a laser’s application? Higher repetition rates offer more pulses per second, allowing for rapid and precise operations.
- Why is thermal management essential in high repetition rate lasers? High repetition rates can generate significant heat, which can degrade performance. Effective thermal management ensures consistent output and extends the laser’s lifespan.
- Are there any other materials suitable for high repetition rate solid-state lasers? Yes, several other materials can be used, but diode crystals and ytterbium-doped crystals are among the most prominent due to their unique properties.
- How does the doping of ytterbium ions enhance a crystal’s properties? Ytterbium ions increase the material’s light amplification properties, making it more efficient for laser applications.