Introduction to Terahertz Generation and its Importance
Terahertz radiation occupies a fascinating middle ground between microwaves and infrared light. Its applications have long been recognized in various scientific and industrial sectors. With the advent of solid-state lasers, we are now experiencing groundbreaking advancements in terahertz generation and its numerous applications. This exploration will delve deeply into the science behind these lasers, their function in producing terahertz waves, and their practical uses in different domains.
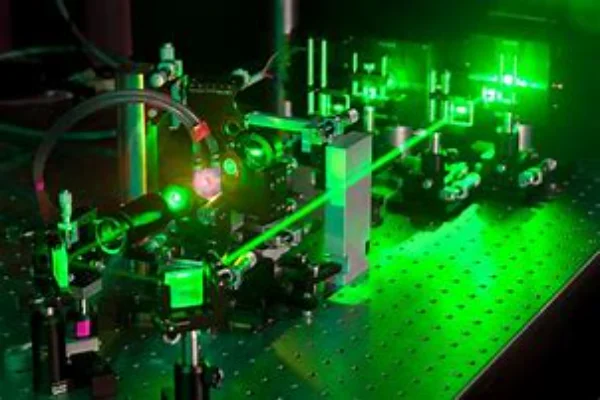
The World of Solid-State Lasers
Solid-state lasers are an integral part of the modern photonics world. Unlike gas or liquid lasers where the active medium is a gas or liquid, solid-state lasers employ solids. The core principle behind these lasers is the use of a host crystal doped with specific ions, known as “lasing” ions. When these ions are excited, they emit photons, leading to the production of a coherent light beam.
One of the most frequently used materials in the creation of these lasers is Nd:YAG, or Neodymium-doped Yttrium Aluminum Garnet. This material’s crystal structure allows for efficient lasing activity when doped with neodymium ions. Another prominent example is the ruby crystal. In ruby lasers, the chromium ions embedded within the aluminum oxide crystal lattice act as the lasing medium. When excited, these ions emit a sharp and intense red light, which was, historically, one of the first demonstrations of laser technology.
Solid-state lasers are renowned for their versatility. Given the array of available host crystals and dopant ions, it is possible to design and engineer these lasers for a wide range of wavelengths and power outputs, making them suitable for a multitude of applications from industrial cutting and welding to delicate medical procedures and, as we’ll delve into further, the generation of terahertz radiation.
Solid-state lasers stand out in the vast landscape of laser technologies. One of their most notable attributes is their efficiency. Because their active medium is solid, these lasers can often achieve higher levels of power conversion efficiency compared to their gas or liquid counterparts. This means that a greater proportion of the input energy is converted into laser light.
Compactness is another distinct advantage. Since they do not require large gas chambers or reservoirs for liquid dye, solid-state lasers can be designed to be much more compact, making them ideal for applications where space is at a premium.
Additionally, these lasers are known for their robustness. Without the need for replenishing gases or liquids, they demand less maintenance and have longer operational lifetimes. Their solid construction also means they are often more resistant to environmental factors, such as temperature fluctuations and mechanical shocks.
Perhaps one of the most exciting characteristics of solid-state lasers, especially in the context of terahertz generation, is their ability to produce high-power, ultra-short pulses. This capacity is crucial for efficiently generating terahertz waves. The high peak powers achieved in these short pulses drive the nonlinear processes that lead to terahertz wave production. As technology evolves, and as our understanding of materials and optics deepens, solid-state lasers will undoubtedly continue to play a pivotal role in driving forward the boundaries of what is possible in the world of photonics.
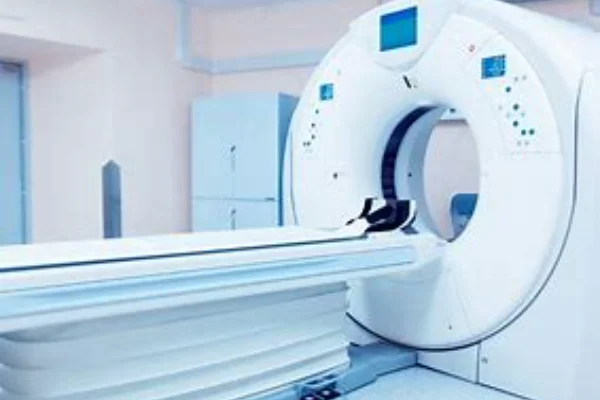
Unraveling Terahertz Generation
Terahertz waves represent an intriguing portion of the electromagnetic spectrum. Sitting between microwaves and infrared radiation, these waves offer a unique blend of properties, with wavelengths that are longer than infrared rays but shorter than microwaves. Because of this unique positioning, terahertz waves open up numerous possibilities, particularly in the realm of spectroscopy.
The power of terahertz radiation has often been compared to the discovery of X-rays in its potential to revolutionize our understanding of materials and processes at the molecular and atomic levels. Imagine being able to “see” through opaque materials or visualize minute changes in a biological cell’s structure. Terahertz waves make these visions increasingly plausible.
Delving into the specifics, one might wonder how exactly these waves are generated. The magic starts with an ultrafast laser pulse. As this pulse, which lasts only a few femtoseconds to picoseconds, interacts with certain materials, it stimulates the emission of electromagnetic radiation in the terahertz range. This process hinges on the principle of nonlinear optics, where the material’s response to the incoming light is not proportional to the light intensity.
It’s worth noting that not just any laser pulse can achieve this feat. The pulse needs to be incredibly short and powerful. Moreover, the material with which the laser interacts must have specific properties conducive to terahertz wave generation.
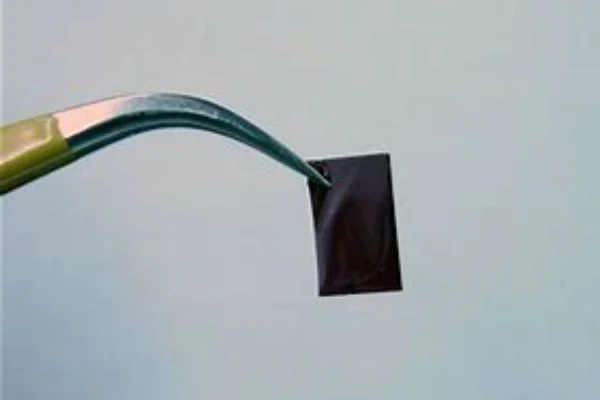
Enter the world of nonlinear crystals. Materials like GaSe and DAST aren’t just ordinary crystals. Their atomic structures and intrinsic properties make them uniquely suited for this purpose. When a powerful ultrafast laser pulse strikes one of these crystals, the nonlinear interaction that ensues can efficiently produce terahertz radiation.
To shed more light on their specific roles: GaSe, with its layered structure, is particularly efficient at generating terahertz waves due to the lack of inversion symmetry in its crystal lattice. This leads to a strong nonlinear response. DAST, on the other hand, has a molecular arrangement that, when subjected to a powerful laser pulse, exhibits strong electron oscillations, effectively converting the laser energy into terahertz radiation.
In essence, while the concept of generating terahertz waves might seem intricate, it’s a harmonious interplay of ultrafast laser pulses and the right materials. With continued advancements in solid-state lasers and a deeper understanding of nonlinear crystals, we stand on the brink of fully harnessing the potential of terahertz radiation for a myriad of applications.
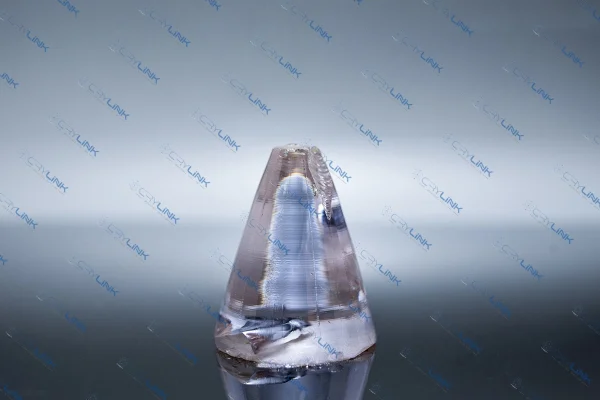
Expanding Avenues in Spectroscopy
Spectroscopy, the study of the interaction between matter and electromagnetic radiation, has long been an invaluable tool in fields as diverse as chemistry, physics, and astronomy. With the emergence of terahertz radiation, the horizons of this technique are expanding even further, providing scientists with a novel tool to peer into the mysteries of various materials. This is not just a minor enhancement; it’s a paradigm shift, reshaping how we approach material analysis and characterization.
Terahertz waves have the unique ability to delve deep into many materials without inflicting damage, a feat not easily achieved by other forms of radiation. This is primarily because these waves have just the right energy levels—enough to probe the material but not so much that they’d cause structural changes or ionization. Their penetration capability, coupled with their distinctive interaction with matter, produces spectral signatures that are often different from those obtained using traditional spectroscopic methods.
The intrinsic characteristics of terahertz radiation position it as an indispensable tool for non-invasive material analysis. The non-ionizing nature of terahertz waves ensures that materials remain unaltered during the investigative process. This quality is of utmost importance when the integrity of the sample is paramount.
Imagine a scenario in the pharmaceutical industry: when developing new drugs, understanding the molecular arrangements and crystal structures of these compounds is vital. Using terahertz spectroscopy, scientists can gain insights into these structures without fear of damaging or altering the samples, ensuring that the data obtained is both accurate and representative of the compound’s natural state.
Similarly, in the realm of art conservation, where priceless artifacts and paintings are studied, the use of non-invasive techniques is crucial. Traditional methods might risk damaging these irreplaceable items. Terahertz radiation can penetrate beneath layers of paint or other coverings, revealing secrets about the artwork’s history, authenticity, or state of conservation—all without causing any harm.
Furthermore, given the specificity of the spectral signatures obtained using terahertz waves, the potential applications extend beyond just identification. They can provide insights into molecular dynamics, chemical interactions, and even the presence of defects or contaminants within materials.
In conclusion, the fusion of terahertz radiation with spectroscopy is not just opening new avenues; it’s broadening the very scope of what’s possible in the world of material science. As we refine our understanding and mastery of this technology, we can expect even more groundbreaking discoveries and applications in the years to come.
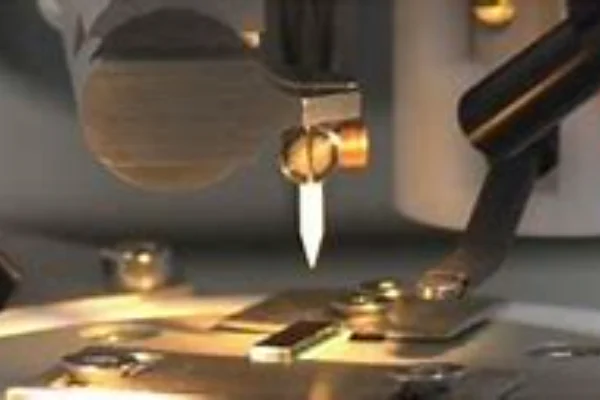
Beyond Spectroscopy – Terahertz in Security and Medical Imaging
The vast potential of terahertz radiation stretches beyond the boundaries of spectroscopy, reshaping industries from security to healthcare. In security screening, terahertz waves offer a transformative approach. Their ability to penetrate clothing and various organic materials, without the ionizing risks of X-rays, allows for the detection of concealed items such as weapons, drugs, and other illicit goods. This ensures heightened security without compromising individual privacy.
Transitioning to the medical arena, terahertz imaging emerges as a cutting-edge diagnostic ally. The distinct interaction of these waves with different tissues provides a precise means to differentiate between healthy and diseased states. Particularly in conditions like skin cancer, terahertz technology offers hope for more effective early detection, paving the way for timely intervention and improved patient outcomes. Together, these advancements underscore the multifaceted applications of terahertz radiation, signaling a new era in both security and medical innovation.
The Future of Terahertz Generation
The relentless advancements in solid-state lasers and nonlinear crystals forecast a bright future for terahertz generation. As technology continues to progress, we can anticipate even broader applications, from wireless communication to novel imaging modalities in the medical field.
Conclusion
The synergy between solid-state lasers and terahertz generation is forging new frontiers in various domains. From deepening our understanding of materials through spectroscopy to revolutionizing medical imaging and security screening, the possibilities are expansive. As we harness these technologies further, the promise of more breakthroughs in the terahertz realm remains undeniable.
FAQs
- What is the significance of the terahertz range?
- The terahertz range sits between microwaves and infrared radiation and has unique properties that allow for deep material penetration without causing damage.
- Why are GaSe and DAST crystals crucial in terahertz generation?
- GaSe and DAST are nonlinear crystals that efficiently produce terahertz radiation when interacting with laser light.
- How does terahertz imaging benefit medical diagnosis?
- Terahertz imaging can distinguish between healthy and diseased tissues, aiding early detection of conditions like skin cancer.
- Are terahertz waves safe for human exposure?
- Yes, terahertz waves are non-ionizing, making them safer for human exposure compared to X-rays.
- How do solid-state lasers differ from other laser types?
- Solid-state lasers utilize a solid gain medium, are compact, robust, and can produce high-power, ultra-short pulses ideal for various applications, including terahertz generation.