In the ever-evolving field of photonics, understanding the thermo-optical properties of crystals stands as a pivotal area of study. These properties, which describe how a crystal’s optical behavior changes with temperature, have profound implications for a myriad of applications, from laser technology to optical communications.
This article dives deep into the world of thermo-optical effects, with a special focus on birefringence and its role in frequency conversion. We’ll also explore the significance of birefringent crystals in wavelength selection and laser frequency conversion.
Birefringence: A Closer Look
Birefringence, a remarkable optical phenomenon also known as double refraction, plays a pivotal role in understanding the behavior of light in crystals. Let’s delve deeper into this fascinating phenomenon and explore its causes and implications.
The Phenomenon of Birefringence
Birefringence is a phenomenon observed when a beam of light enters a crystal and is split into two refracted rays. This occurs because the crystal possesses two distinct refractive indices for light polarized in two different directions. As a result, the two refracted rays travel at different speeds through the crystal, leading to a phase difference between them.
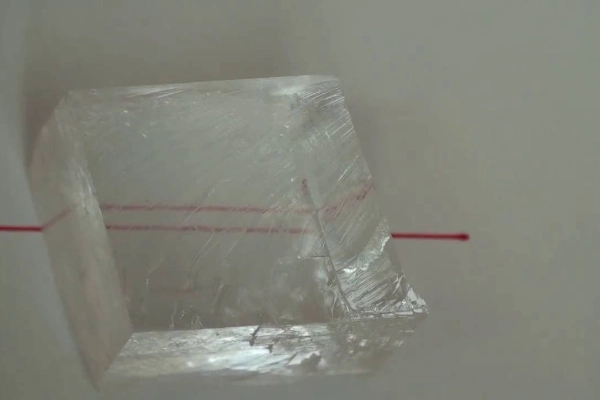
The phase difference introduced by birefringence can have profound effects on the polarization state of light passing through the crystal. Depending on the orientation and properties of the crystal, the emerging light may exhibit linear or circular polarization, or it may experience a shift in phase that can be harnessed for various applications.
Birefringence is a fundamental concept in optics and crystallography, with broad applications in fields ranging from geology and materials science to microscopy and telecommunications.
Causes of Birefringence
Several factors can give rise to birefringence in crystals, each contributing to the phenomenon in unique ways:
- Structural Birefringence: This type of birefringence is rooted in the inherent anisotropy of a crystal’s lattice structure. Crystals possess different optical properties along different crystallographic axes. As light travels through the crystal, it interacts with these varying properties, resulting in the splitting of the incident beam.
- Stress-induced Birefringence: External mechanical stresses applied to a crystal can induce birefringence. Stress alters the crystal’s refractive indices, causing them to differ for light polarized in different directions. This type of birefringence is often observed in transparent materials like glass under pressure or strain.
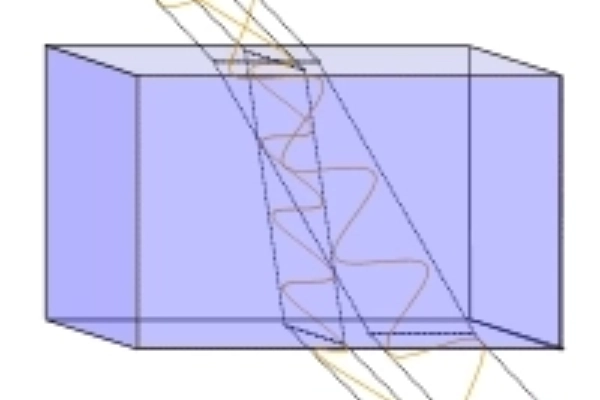
- Thermo-optic Birefringence: Temperature gradients within a crystal can lead to birefringence. Variations in temperature affect the crystal’s refractive indices, creating a mismatch for light with different polarizations. Thermo-optic birefringence is particularly relevant in crystals used in high-temperature environments or laser applications.
Understanding the causes of birefringence is essential for controlling and mitigating its effects in practical applications. Engineers and scientists utilize this knowledge to design optical devices, correct for birefringence-induced distortions, and develop materials with tailored optical properties. Birefringence’s ability to manipulate the polarization and phase of light makes it a valuable tool in modern optics and photonics.
Phase Matching for Frequency Conversion
Phase matching, a fundamental technique in nonlinear optics, is of paramount importance, especially in optimizing processes like frequency conversion within birefringent crystals. Let’s delve deeper into the complexities of phase matching and its profound significance within the domain of optics.
Phase matching serves as a linchpin for numerous optical applications, ensuring efficient energy transfer and enabling the generation of specific wavelengths with remarkable precision. This technique holds the key to advancing technologies in fields ranging from telecommunications and spectroscopy to quantum computing and medical imaging.
Understanding Phase Matching
Phase matching is a method employed to maximize the efficiency of nonlinear optical processes by ensuring that interacting light waves remain in phase over a significant interaction length. When two or more waves interact within a nonlinear crystal, they can either constructively or destructively interfere, depending on their relative phases.
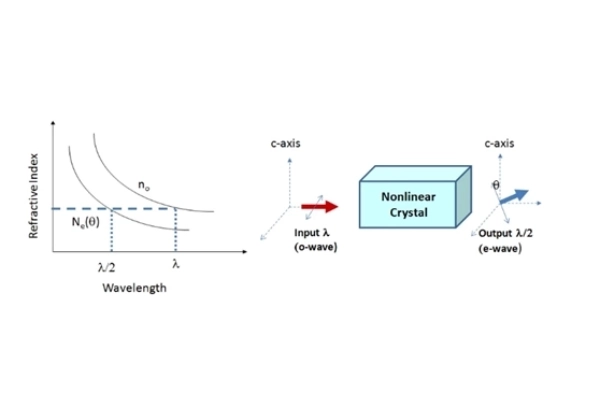
In the context of frequency conversion, such as second harmonic generation, phase matching is crucial to ensure that the incident light wave at one frequency efficiently generates a new wave at a different, desired frequency. The objective is to align the phase velocities of the interacting waves so that they reinforce each other, leading to optimal energy transfer and efficient conversion.
Achieving Phase Matching
Phase matching can be achieved through various techniques:
- Adjusting Crystal Orientation: One common approach is to adjust the orientation of the birefringent crystal relative to the incident light. By carefully choosing the crystal’s orientation, it is possible to make the refractive indices for the interacting waves equal, ensuring that they propagate at the same phase velocity. This alignment maximizes phase matching and, consequently, the conversion efficiency.
- Temperature Tuning: Another method involves changing the crystal’s temperature. Temperature fluctuations can alter the crystal’s refractive indices, providing a means to fine-tune the phase matching conditions. By carefully controlling the crystal’s temperature, researchers can optimize phase matching for specific nonlinear processes.
Role of Birefringent Crystals
Birefringent crystals are invaluable components in various optical applications, and their unique properties make them indispensable in the field of optics.
Wavelength Selectors
Birefringent crystals find essential roles in wavelength selectors due to their ability to separate incident light into its constituent wavelengths based on polarization. By adjusting the crystal’s orientation, specific wavelengths can be selected or filtered out, making these crystals critical tools in spectroscopy and other optical applications. Birefringent crystals enable researchers to precisely control which wavelengths are allowed to pass through, facilitating detailed spectral analysis.
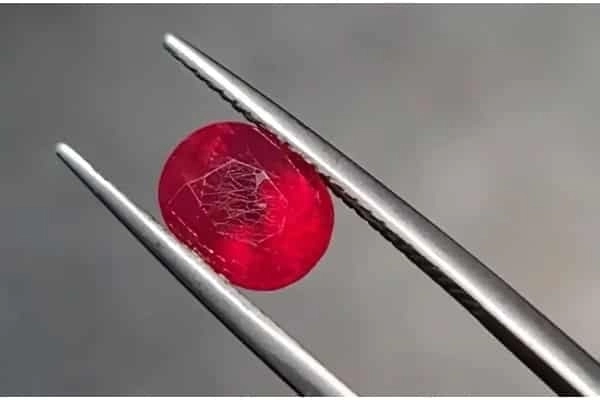
Laser Frequency Conversion
In laser systems, birefringent crystals are key players in frequency conversion processes, such as second harmonic generation (SHG) and parametric oscillation. SHG, for example, involves the efficient conversion of a laser beam at one frequency into a beam at twice the frequency. Birefringent crystals play a crucial role in phase matching for these processes, ensuring that the interacting waves are in phase and enabling efficient frequency conversion. This capability is instrumental in generating laser light at specific wavelengths required for various applications, from medical treatments to telecommunications.
Advancements in Thermo-optical Research
With the rapid advancements in materials science and optics, researchers are continuously uncovering new thermo-optical effects and exploring innovative ways to harness them. From the development of novel birefringent materials with tailored properties to the optimization of phase matching techniques for enhanced efficiency, the future of thermo-optical research holds great promise.
As technology evolves, the precise control of optical processes, including phase matching in birefringent crystals, will continue to drive innovations in fields like photonics, quantum optics, and materials science. These advancements will contribute to the development of more efficient and versatile optical devices with applications ranging from data communication to cutting-edge scientific research.
Conclusion
In conclusion, the thermo-optical properties of crystals, with a specific focus on birefringence, play a pivotal role in a wide array of optical applications and technologies. These properties are not only fundamental to our understanding of light-matter interactions but also serve as the cornerstone of many cutting-edge advancements in photonics.
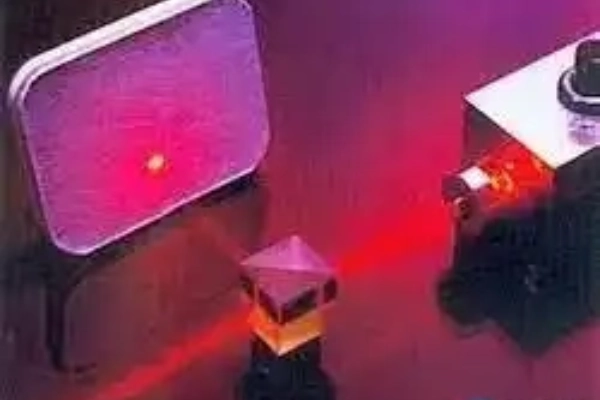
As we delve deeper into the intricacies of these optical effects, we gain the knowledge and tools necessary to harness them effectively. This understanding is essential for the continued evolution of optical systems that are more efficient, versatile, and powerful than ever before.
The ongoing research in materials science and optics promises to unveil new facets of thermo-optical behavior, leading to the development of novel materials and innovative techniques. These advancements will undoubtedly have a transformative impact across numerous fields, from telecommunications and data processing to medical diagnostics and quantum technologies.
In essence, our exploration of the thermo-optical properties of crystals is not just a journey into the fascinating world of optics; it’s a journey towards unlocking the full potential of light-based technologies that will shape the future of science and industry.
FAQs
- What is the primary cause of birefringence in crystals?
- Birefringence primarily arises due to the anisotropic nature of a crystal’s lattice structure, leading to different refractive indices for different polarization directions of light.
- How does temperature affect birefringence?
- Temperature can induce changes in a crystal’s refractive indices, leading to thermo-optic birefringence. Additionally, temperature can be used to achieve phase matching in nonlinear optical processes.
- Why is phase matching important in frequency conversion?
- Phase matching ensures that the interacting light waves remain in phase over their interaction length, maximizing the efficiency of energy transfer between them.
- Can all crystals exhibit birefringence?
- Not all crystals exhibit birefringence. The effect is prominent in crystals with anisotropic lattice structures, such as calcite and quartz.
- What are the applications of birefringent crystals beyond lasers?
- Apart from lasers, birefringent crystals find applications in optical instruments, spectroscopy, and telecommunications, among others.